Building evidence —
A mutation that’s becoming common seems to boost infectivity in cultured cells.
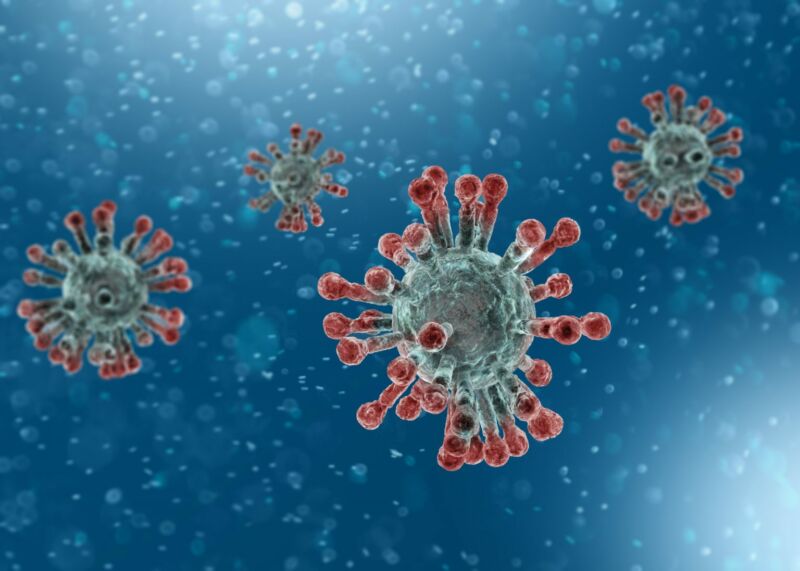
About a month ago, news reports painted a potentially grim picture: a single mutation in SARS-CoV-2 was taking over the world, rapidly displacing earlier forms of the virus in most locations. The researchers behind the finding suggested we were watching in real time as SARS-CoV-2 was evolving into a form that spreads more readily within human populations.
While the evidence was suggestive, it wasn’t decisive, and there were a number of alternative explanations for the pattern of viral spread. As is so often the case in science, we needed more data. Now, some additional data has arrived in the form of a draft paper that suggests that there’s a biochemical reason for why the mutated form of the virus might be more potent.
Scientific back and forth
The idea behind the original results was fairly simple. If we assume that mutations show up at random in the coronavirus genome, then the chance of two identical mutations appearing independently in the virus’s genome is pretty low, given that the genome is over 30,000 bases long. Thus, if we see the same mutation in two different locations, the chances are very good that they got there through common descent from an ancestor that had the mutation.
The researchers found that not only was a single mutation showing up in lots of different places, but it was becoming the dominant form in most of them. Given the assumption of a single origin, this suggests that one mutant form of the virus is not only better than most others in spreading to new locations, but it’s displacing other strains once it gets there. This, to the authors, led to an obvious conclusion: the mutation conveyed some sort of selective advantage to the virus, possibly allowing it to replicate or spread more readily.
But some results that came out shortly after the draft was posted raised questions about the study—not its data, but the key assumption behind it. A study published in Science Advances found that our cells’ RNA-editing system, which chemically alters specific RNA sequences, is active against the genomes of coronaviruses. While we don’t know whether the system will edit the sequence that differs between the mutant form and the ancestral virus, the finding suggests that certain mutations in the viral genome are likely to happen multiple times.
If a mutation is likely to arise multiple times, then common descent can’t be assumed. And without common descent, it’s difficult to say whether the pattern observed in the global data is the result of selection or not.
Biochemistry steps in
But the whole evolutionary argument is a way of trying to identify signs of whether this mutation alters the behavior of the virus. It’s possible to just directly look at whether the mutation changes some aspects of the virus, and that’s what the new results are all about.
The mutation alters the sequence of the spike protein, which sits on the surface of the virus and latches on to a protein on the surface of human cells and then fuses the virus’ membrane with the cell’s, allowing the virus to enter the cell and begin a new infection. So it could very easily alter the dynamics of the pandemic by influencing how readily mutant viruses enter cells. To find out more, the researchers engineered the spike protein—both the ancestral and mutant form—into a harmless virus and tested how well it infected cells in culture. The mutant form got into the cells at a rate that was nine times higher than the ancestral form.
How could that work? The answer depends on some fairly obscure viral biology. To function, the spike protein has to be split into two halves, which continue to associate even after they are no longer chemically linked. In most coronaviruses, the splitting takes place after the virus is on the surface of the cell it will infect and is catalyzed by protein-cutting enzymes made by that cell. SARS-CoV-2 is different in that the split is made in the cell the virus originates in. There’s a specific site in the spike protein of SARS-CoV-2 that’s recognized by a different group of protein-cutting enzymes; as far as we know, this site is distinct to the virus, though it may eventually turn up in viruses from other species.
The result of this is the SARS-CoV-2 spike protein’s halves have to remain associated while the virus travels to new cells and infects them. Since other coronaviruses have spike proteins in which these halves remain chemically linked during this process, it doesn’t matter how tightly the halves associate. But for SARS-CoV-2, the strength of their association is likely to be critical. The researchers therefore hypothesized that the mutation strengthened this association.
To test this, they put tags on both ends of the spike protein and then allowed it to be cut in half. After the cut, the halves of the mutant protein were found to stay together at a much higher rate than the original form—different experiments gave different values, but the difference ranged roughly from twofold to fivefold more. This result also held true for when the protein was incorporated into a harmless virus.
More sticking power
The result of this increased stability is an increase in the virus’s ability to latch onto the protein it targets on the surface of human cells. This is true even though the mutant form of spike doesn’t have an increased affinity for its target protein; there’s simply more of the functional form around.
The study comes with some very important caveats: one, this work wasn’t done with an intact coronavirus. Instead, it was done by putting key coronavirus proteins into a different (and harmless) virus. Second, and more critically, this work wasn’t done in living organisms, but in cells. There’s a lot going on inside the body that makes it a very different environment from the layer of warm liquid that cultured cells live in. And as with all draft papers, keep in mind that peer review may cause the final, published results to look significantly different from the ones here.
Those caveats handled, the evidence does seem to be tending toward this mutation making a difference in the virus’s spread. We’ve gone from having a suggestive pattern of genetics to having a reasonable hypothesis about the mechanism underlying them and some biochemical support for that mechanism. Even if none of these bits of evidence is strong enough to make the case decisive, there’s beginning to be some weight behind the idea.